NOTE: Some content may not display correctly, including tables and figures. See PDF for full details.
A Strategy for Growth of Electricity Generation in India
India is the largest democracy with nearly one-sixth of world population and low per capita income. In recent years, it has witnessed an impressive growth rate in GDP. Development aspirations of its populace demand that this growth rate be sustained for a long enough time so as to enable them to have decent enough quality of life. This necessitates a matching growth in the availability of energy. Further, the development process, is also driving, as expected, a shift in energy use from non-commercial energy sources to commercial sources, particularly electricity. This phenomenon is similar to what has been witnessed by the developed countries in the west. But the situation in India is more complex because of high density of population, continuing growth of population and demographic shift from rural to urban areas.
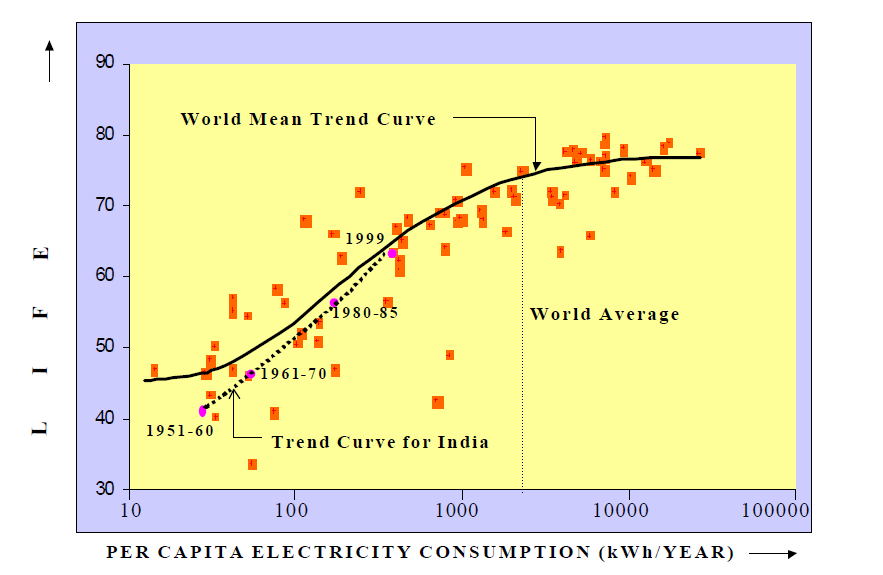
PER CAPITA ELECTRICITY CONSUMPTION (kWh/YEAR)
Electricity is the main driving force for development in today’s industrial world. Per capita gross national product is directly related to the per capita electricity production in the country. Analysis of statistics also indicates that per capita electricity consumption is directly related to life expectancy. If one plots per capita consumption of electricity on a logarithmic scale against life expectancy on a linear scale, one gets a very interesting S- shaped curve. India is right in the middle of the linear portion with a life expectancy of about 63 years now. This means that any additional electricity generation in the country will lead to a sharp increase in life expectancy. With increased availability of electricity, smaller towns and villages will have safe drinking water, better sewage treatment facilities and better primary health care and all these have a very beneficial impact on all health parameters and on the ultimate health parameter, viz. life expectancy.
Let us look at some statistics. The electricity generation in the fiscal year 2001-02 was about 515 billion kWhr from electric utilities1 and additional about 120 billion kWhr were generated by the captive power plants2. On per capita basis, this works out to 610 kWhr per year. In the countries comprising Organisation for Economic Cooperation & Development (OECD), the corresponding figure is about 10,000 kWhr3. If we consider that continuously improving energy intensity of GDP and the fact that India has a tropical climate, we would need per capita electricity generation at a modest level of about 5000 kWhr per year to reach the status of a developed nation. India’s population could rise to 1.5 billion by the year 2050. Therefore, the country has to plan to have total electricity generation of about 7500 billion kWhr per year by the year 2050. This is about 12 times the generation in the fiscal year 2001-02. Electricity generation of this magnitude calls for a careful examination of all issues related to sustainability including abundance of available energy resources, diversity of sources of energy supply and technologies, security of supplies, self sufficiency, security of energy infrastructure, effect on local, regional and global environment and demand side management.
The concept of sustainability calls for exploitation of available resources to improve the quality of life of people without harming the interest of future generations, both from the point of availability of resources as well as degradation of environment beyond the inherent corrective capability of natural processes. While environmental burden has to be kept within the limits of self-correction and geographically well distributed, development aspirations of people have to be given a place of supreme importance in all decision making processes. After all “poverty is the biggest polluter” and is the source of several conflicts.
While sustainable development is a commonly heard term, what is forgotten is that sustainable development requires deployment of relevant technological options and this can come about only by adopting strategies for development of relevant technologies. This is not an easy task and requires long-range planning and that is what we have done in the Department of Atomic Energy (DAE) in India. We have adopted a strategy for achieving a high degree of self-reliance by carrying out research and technology development in a wide range of disciplines relevant to exploitation of indigenous nuclear energy resources. 1 Personal communication, CEA, May 2002.
2 PowerLine November 2002, page 10. This is based on the assumption that all captive power plants (Recognized and unrecognized) operated at a capacity factor of 50% in the fiscal year 2001-02.
3 World Energy Outlook – 2000 – Highlights, page 48, International Energy Agency, Paris.
Let us examine the fuel resource situation in India (Table 1). We have reasonable coal reserves – about 221 billion tonnes (Proven: 84, Indicated 99 and Inferred: 38). India is the third largest coal producing country in the world and more than 70% of our electricity is produced by coal based thermal power plants. Following the procedure of assigning reserves with 90% confidence level to the proven category, 70% to the indicated category and 40% to the inferred category and then applying the criterion of reserve to extraction ratio of 4.7:1, the working group on coal and lignite for the Xth five year plan has tentatively projected the extractable coal to be only 37.86 BT. During 2000-01, the domestic production of coal was 310 MT, while imports were 23.3 MT. Considering our current dependence on coal for energy supplies and projected requirements, one can calculate how long domestic coal resources are going to last and estimates by us in DAE and also by some other agencies in the country indicate that we will have difficulties with regard to availability of coal by the middle of the present century or even earlier. In addition, coal based stations are likely to pose serious problems in future arising out of transport of large quantities of coal across the country and environmental problems related to disposal of ash and emission of greenhouse gases and acid gases. Our oil and natural gas reserves are very modest and we are importing a very
significant | share of our requirements which constitutes a major | share of our overall |
imports. | Hydro-potential is renewable and must be exploited to | the maximum, but a |
significant | portion of our hydro-potential is concentrated in the | north-east, where its |
exploitation is problematic because of a variety of reasons including political situation, difficult terrain and distance from electricity demand centres. In general, exploitation of hydro-resources is handicapped by issues like displacement of people and possible effects on ecology. Non-conventional sources like solar, biomass and wind will play useful roles, but at the present level of technology development they can only complement electricity generation by base load stations based on fossil, hydro or nuclear plants.
Our uranium deposits are limited, while thorium deposits are large. Uranium-238, the dominant isotope of uranium is a fertile material and cannot make a reactor critical by
4 Key world energy statistics, International Energy Agency, 2002 edition, page15.
itself. It has to be converted to fissile plutonium-239. The process of conversion takes place in a nuclear reactor and spent fuel from thermal reactors contains plutonium-239. On discharge from the reactor, spent fuel can be dealt with in two ways. The first one termed ‘open cycle’, consists of treating the entire spent fuel as waste and disposing it as such. With this approach only a small fraction of the energy potential exploitable from uranium is utilised. To avoid this colossal waste, a closed fuel cycle involving reprocessing of spent fuel to separate plutonium and uranium-238 has to be pursued. Besides recovering valuable fissile material, reprocessing helps to sort out the wastes according to their activity levels and their decay period thereby assisting waste disposal and minimising environmental impact. Similarly thorium is a fertile material and has to be converted to a fissile material viz. Uranium-233. To ensure long term energy security for the country, we have chosen to follow a ‘closed cycle’ approach. Pursuit of the closed cycle approach calls for setting up of reprocessing plants and breeder reactors. Closed cycle has the capability to virtually de-couple energy supply from resource related constraints for generations to come
We, in India, have taken cognisance of these facts viz. our resource position and need for ensuring long-term energy security and accordingly formulated a three-stage nuclear power programme. The first stage comprising setting up of Pressurised Heavy Water Reactors (PHWRs) and associated fuel cycle facilities is already in the industrial domain. The speed at which our nuclear power programme can move forward is no longer limited by technology or the country’s industrial infrastructure, but by the availability of funds. At present, we have 12 such reactors in operation and six under construction, which include indigenously designed and developed 540 MWe units under construction at Tarapur. The designs of these reactors have progressively evolved taking into account the needs for indigenisation, our own operating experience, operating experience in PHWRs outside the country and progressive evolution of enhanced safety features. We are now self sufficient in all aspects of PHWR technology. As we gain experience and master various aspects of the nuclear technology, performance of our plants is also improving. Average capacity factor of our plants have steadily risen from 60% in 1995-96, to about 90% in the year 2002-03. Nuclear power plants have so far produced 200 billion units. We have accumulated about 200 reactor-years of operational experience free of any serious incident involving release of radioactivity to the environment.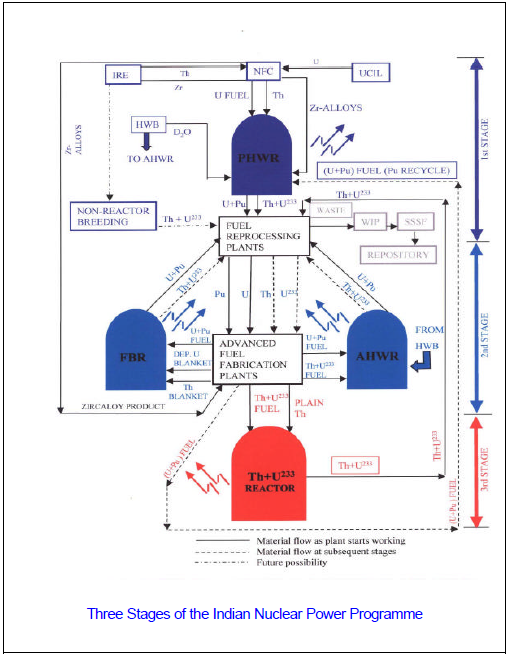
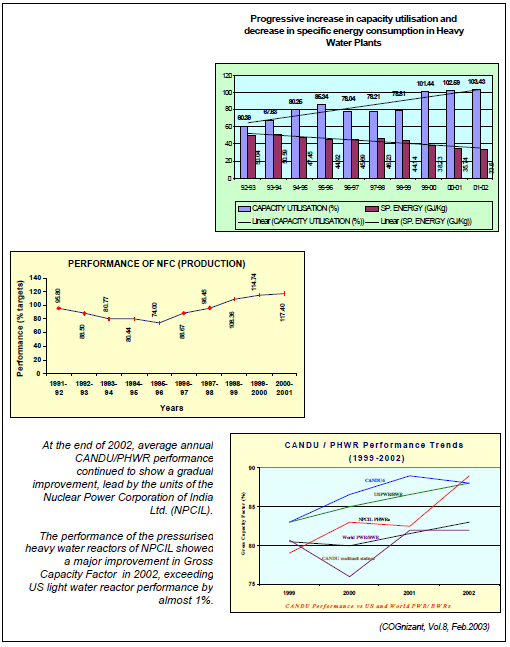
KalpakkamReprocessingPlant,Kalpakkam,(TamilNadu)
achieved all its technology objectives. Its fuel has crossed a burn up of 100,000 MWd/tonne, a value four time larger than the original design value. Based on the experience gained with this reactor and with active cooperation of academia and industry detailed design and technology development of the 500 MWe Prototype Fast Breeder Reactor (PFBR) has been completed and we are processing financial and administrative approvals to start its construction. Pre-project activities for this project have already commenced at Kalpakkam near Chennai.
Performance of Indian Nuclear Power Plants
Schematic of Prototype Fast Breeder Reactor
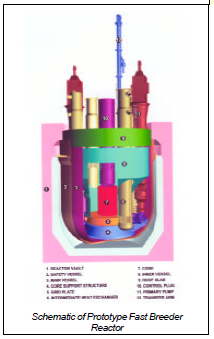
The third stage will be based on the thorium- uranium-233 cycle. Timely implementation of a programme for thorium utilisation in our programme is very crucial to meet the increasing energy demands in the country. A small beginning has already been made by introducing thorium in a limited way in research reactors and in PHWRs. With sustained efforts over the past several years, we have small scale experience over the entire thorium fuel cycle. A research reactor KAMINI is operating in Kalpakkam based on Uranium-233 fuel which is derived from thorium. This fuel was bred, reprocessed and fabricated indigenously. An Advanced Heavy Water Reactor (AHWR) has now
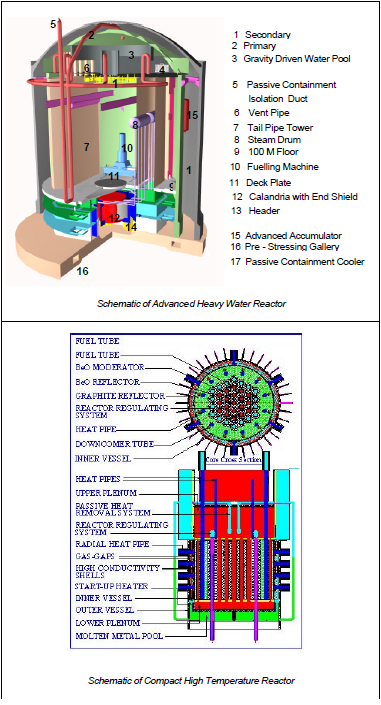
been developed at the Bhabha Atomic Research Centre (BARC) to expedite transition to thorium based systems. The reactor physics design of AHWR is tuned to generate about 65% power from thorium and incorporates several advanced safety features. The detailed project report of this reactor has been prepared and is undergoing a structured peer review before we launch its construction some time in 2004-05.
As a next step towards self sustained thorium utilization with a potential for growth, a road map for the development of Accelerator Driven System (ADS) has been prepared. In the ADS, high energy proton beam generates neutrons directly through spallation reaction in a non-fertile/ non-fissile element like lead. A sub-critical blanket can then further amplify this external neutron source as well as produce energy. Development of such a system offers the promise of shorter doubling time with thorium-uranium-233 systems, incineration of long lived actinides and fission products and can provide a robust technology base to large scale thorium utilization. As a first step towards realization of ADS, we are launching development of proton accelerator in the X five year plan.
To jump start the nuclear power programme, two Boiling Water Reactors were set-up at Tarapur near Mumbai. These reactors are still in operation with an impressive record of performance in recent years. In a similar manner, as an additionality to the indigenous self- reliant three-stage programme, we are looking for likely sources for import of light water reactor technology. Such imports have to conform to the latest safety standards and should be economically attractive. The deal with the Russian Federation for setting up 2 x 1000 MW units at Kudankulam is a step in this direction. Overall, we plan to have an installed nuclear capacity of about 20,000 MW by the year 2020 and this would include some plants based on advanced LWR technology (Table 2).
It is worthwhile to compare import of nuclear fuel with the import of other forms of fuel. Nuclear fuel contains energy in a concentrated form thus requiring much less tonnage for fuel to be transported or stored. In the overall cost of electricity generated from nuclear fuel, the cost of fuel is a much smaller component as compared to the other components. This can be seen by the data in the Table 3. Further, the fuel discharged from a nuclear reactor can be reprocessed to recover plutonium, which can then be used in fast reactors to produce electricity. The cost quoted in the Table 3 assumes that the fuel is used in once through cycle and if one accounts for the recycle option, this would reduce by a factor of about 60. One may dispute the accuracy of these numbers, but one cannot dispute the order of magnitude difference between the characteristics of nuclear fuel and other fuels. Thus, if import of energy is a necessity, from strategic considerations nuclear fuel is a preferable option.
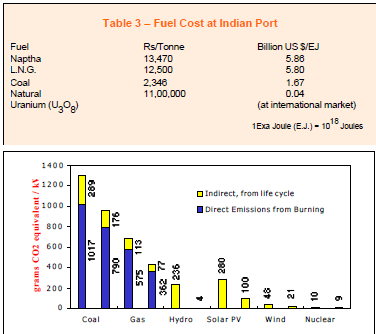
The comparative economics of nuclear power plants depends on local conditions, discount rates and availability of cheap fuels like coal and gas. Wherever fossil fuels are available at reasonable prices, the setting up of thermal power plants is an option to be considered in any techno-economic analysis. Issues to be considered in case of thermal plants include location of coalmines vis-a-vis load centres, coal transportation, availability of railroads for transportation, sulphur and ash content of the fuel and associated environmental impact. Hydropower provides low cost electricity generation, but sites available for large projects are limited and social impact is very high due to submergence of large areas. Gas prices are subject to fluctuations due to market forces and form a sizable fraction of electricity cost produced from gas fired plants. Therefore, the cost of electricity generated from gas fired plants can vary a lot depending on the market conditions.
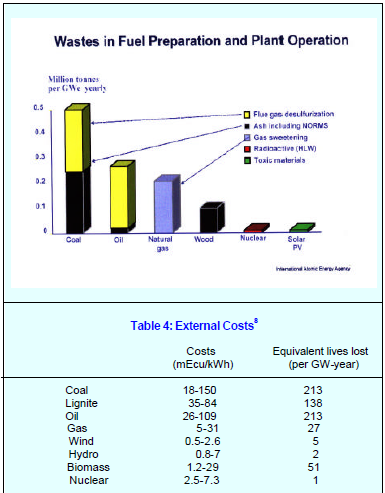
|
Table4:ExternalCosts Costs (mEcu/kWh) | 8 Equivalent lives lost (per GW-year) |
Coal | 18-150 | 213 |
Lignite | 35-84 | 138 |
Oil | 26-109 | 213 |
Gas | 5-31 | 27 |
Wind | 0.5-2.6 | 5 |
Hydro | 0.8-7 | 2 |
Biomass | 1.2-29 | 51 |
Nuclear | 2.5-7.3 | 1 |
Generally only direct costs are used in comparative assessment of different electricity options. However, the opinion is building up in favour of internalising all costs of generation in any comparative assessment of energy options and this would include, inter alia, the cost of impact on environment and health and cost of setting up of infrastructure for fuel transportation which is often subsidised. The largest environmental impacts associated with fossil fuels are carbon dioxide and other forms of air pollution which can cause chronic illness. The risks associated with these impacts affect the entire planet. In addition, the volume of waste generated in case of energy generation from fossil fuels is quite large. Technically nuclear energy is far more benign and much of the cost is already internalized in financial plans. For example, nuclear power operators are required to provide funds for decommissioning of installations. External costs have been estimated by a study conducted under European Commission’s ExternE project results and reported in 1998 are summarized in the Table 4.
8 Adapted from European’s Commission’s ExternE project - 1998
An internal study done by Nuclear Power Corporation of India Ltd. (NPCIL) indicates that nuclear power is competitive compared to coal fired thermal power, when the nuclear plant is about 1000 km from the pit-head. There are several regions in the country where such haulage is involved. Being capital intensive in nature, the cost of nuclear electricity becomes more competitive with the age of the plant as the capital cost depreciates. Interest during construction (IDC) adds to capital cost of a plant and it is necessary to reduce the gestation period. NPCIL is working towards reducing the gestation period by adopting innovative project management practices including having large EPC packages and the plants under construction will be completed in about 5 years.
Carbondioxide(CO ) Emission per capita in tonnes / year 2 |
Party Emission %age of World Population | %Share of World Total |
OECD 10.9 18.6 | 49.6 |
China 2.5 21 | 12.8 |
India 1.1 16.6 | 4.4 |
All Developing | |
countries 1.9 77.5 | 35.8 |
Least Developing | |
Countries 0.2 10.4 | 0.4 |
World 4.1 - | - |
HumanDevelopmentReport2002 | |
To reduce the risk of global climate change, industrialized countries have made commitments to reduce GHG emissions under a protocol, negotiated in Kyoto, Japan in 1997 as an addition to the 1992 United Nations Framework Convention on Climate Change (UNFCCC). In the so-called Kyoto Protocol, industrialized countries have agreed to reduce their collective emissions during the period 2008-2012 by at least 5.2% below 1990 levels. So far no decision has been taken about carbon reduction commitments for the period beyond 2012, but statements have already been made, that countries like India and China should also make carbon reduction commitments. It is pertinent to note that per capita carbon emission in India is 1.1 tonne per year, it is 2.5 tonnes per year in China while for the OECD countries, it is 10.9 tonnes per year. Therefore, while negotiating at international fora we have to take a careful stand with regard to accepting any carbon reduction commitments. At the same time, we have to adopt policies wherein we use advanced technologies so as to minimize carbon emissions. As far as energy is concerned, nuclear energy produces virtually no GHG emissions and should be an important part of our strategy to reduce GHG emissions.
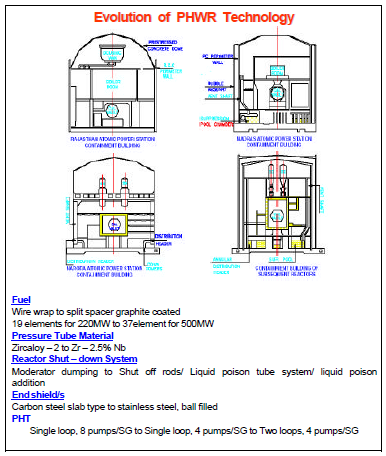
Since the very inception, the Indian nuclear power programme has laid a strong emphasis on nuclear safety and radiation surveillance in the environment. The essence of our safety
policy was spelt out by Dr Bhabha5 , when he said, “Radioactive materials and source of radiation should be handled in a manner which not only ensures that no harm can come to workers or anyone else, but in an exemplary manner so as to set a standard which other organisations in the country may be asked to emulate.” This has really happened. In all industrial development programmes in India, environment monitoring has assumed importance and statutory regulations have been established. Systematic measurements of radiation levels in the environment and related investigations have been undertaken by the DAE long before such regulations were introduced.
Concern for safety pervades all aspects of the nuclear fuel cycle, in the design and engineering, in plant operation, and in regulation of the nuclear industry as a whole. In design and engineering, this includes adopting sound basic designs, adhering to appropriate codes and practices, using fully tested materials and components, providing adequate margins and failsafe arrangements, and facilitating maintenance and proper operation. Design of PHWRs in India has progressively evolved by our efforts in a manner that all attempts have been made to improve plant safety. An example is the manner in which reactor containment has evolved from the reactor near Kota (RAPS) to those at Kaiga. At RAPS we have a dousing tank, at Kalpakkam (MAPS) vapour suppression pool was introduced, at Narora partial double containment was introduced, and at Kakrapar full double containment was incorporated. At Kaiga, for the first time a high strength concrete has been used for the containment dome. In a similar manner, other systems such as secondary shut down system, emergency core cooling system have been evolved so as to ensure plant safety under all anticipated operating conditions.
5 Dr Homi J Bhabha, The first Chairman of Atomic Energy Commission, India
As indicated earlier, we have designed an Advanced Heavy Water Reactor, which besides using thorium, also aims at enhancing safety to a level far higher than current safety requirements, which themselves are very stringent. Examples of safety features include pumpless primary cooling system, operator forgiving characteristics with a grace period of three days, passive containment isolation, elimination of impact in public domain etc.
Atomic Energy Regulatory Board (AERB), an independent regulatory body in the country, is responsible for licensing and regulation of all activities related to atomic energy. To provide fillip to regulatory R&D, AERB has set up an independent Safety Research Institute (SRI) in 1998 at the IGCAR campus in Kalpakkam. It is an ideal site as it houses a range of fuel cycle facilities like PHWRs, a fast reactor, a Uranium-233 fuelled reactor, fuel reprocessing plants, waste management facilities and several research laboratories. SRI conducts a substantial part of the research programme in an inter-institution manner. Areas of research covered by SRI include nuclear plant safety, radiological and environmental safety, fire and industrial safety.
With total protection of the environment as the overriding consideration, management of the radioactive waste generated in the fuel cycle has received high priority in our nuclear programme right from its inception. Based on indigenous materials and capabilities, technology has been developed and is in routine use for the management of low and intermediate level wastes meeting the stringent regulatory requirements and standards. No waste in any physical form is released to the environment unless the same is well below internationally accepted safe levels. Treatment of reprocessing waste has received considerable attention because they contain nearly 99% of the activity generated in the nuclear fuel cycle. Based on years of development studies, a long-term action plan has been formulated for the management of these wastes.
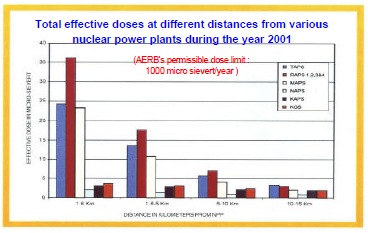
In principle the Indian programme envisages two distinct modes of final disposition in respect of radioactive wastes:
Near surface engineered extended storage for low and intermediate level wastes.
Deep geological disposal for high level wastes and alpha bearing wastes.
A waste immobilisation plant for the treatment of High Level Waste (HLW) has been operational at Tarapur for quite some time and one more has been just commissioned at Trombay. One more waste immobilisation plant is being set up at Kalpakkam. Use of Joule heated ceramic melters for vitrification is under development. A solid storage and surveillance facility (SSSF) has also been set up for interim storage of vitrified HLW.
As regards ultimate disposal, considering the present small size of the Indian programme, considerable time is available before the need for a repository would be felt. However, studies on establishing a repository are being carried out in an ongoing manner. An experimental research station was set up in an unused portion of an underground mine located at Kolar near Bangalore. In-situ experiments for examining the thermal, mechanical, hydrological and chemical behaviour of the host rock under simulated conditions have been conducted.
High level waste disposal is often cited as show stopper for nuclear power. However, technological solutions for long-term storage of high level waste do exist now and what remains is implementation. Several countries have taken steps in this direction. Yucca mountain, Nevada has been approved as the site for national repository for nuclear fuel and high-level radioactive waste in the USA6 . Finland has also gone ahead with legislative approval for setting up a rock characterization facility at Olkiluto power station7 . Further R&D in waste transmutation should soon make high level waste storage a short term issue.
6 NucNet News No 254/02/A, 24 July 2002
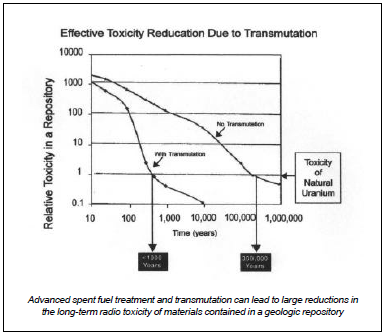
India has above 16% of the world’s population and only about 6% of the world’s coal reserves, oil and gas being less than 1%. However, India has about 32% of the world’s Thorium reserves. We have large hydro potential, but its exploitation is beset with issues like displacement of people and possible effects on ecology. Non-conventional sources, at present stage of technology development, are suited essentially for decentralised small capacity plants. Large-scale development of nuclear power is thus inevitable. Based on in-house discussions, we have set a target to have an installed capacity of about 20,000 MWe by the year 2020. Many consider this target to be modest and realisable. India has developed comprehensive capabilities in all aspects of nuclear fuel cycle and as far as nuclear technology is concerned, India is a “developed country”.
We believe that nuclear power is a clean source of energy. However, to make nuclear power economically more competitive, nuclear Industry has to continue to improve existing technologies, develop new technologies and adopt innovative project management practices to reduce capital cost and shorten construction period.
7 Nucleonics Week, May 24, 2001, p1